1. Scientific Rationale
1.1 COMPETING GEOMETRIES
Determination of the geometry of inner regions of accreting BH (BH) sources is obviously of major importance for our understanding of the physics of those sources. There are now, in particular, a large number of models of the X-ray emission of these sources, explaining it in terms of accretion discs and their coronae, hot inflows/outflows, and jets. The two main types of those sources are AGNs and accreting X-ray binaries (XRBs) containing BHs. Here, we concentrate on the latter.
Luminous BH XRBs show two main spectral states, hard and soft. There is a relative consensus regarding the geometry in the soft state. The main component of the accretion flow is an optically-thick and geometrically-thin accretion disc (Shakura & Sunyaev 1973; Novikov & Thorne 1973) extending down to the innermost stable circular orbit (ISCO). The hard X-ray tails present in this state with various relative amplitudes are probably due to coronal emission containing a hybrid (i.e. both thermal and non-thermal) distribution of electrons Compton up-scattering disc blackbody photons (e.g. Gierliński et al. 1999; Poutanen & Vurm 2009).
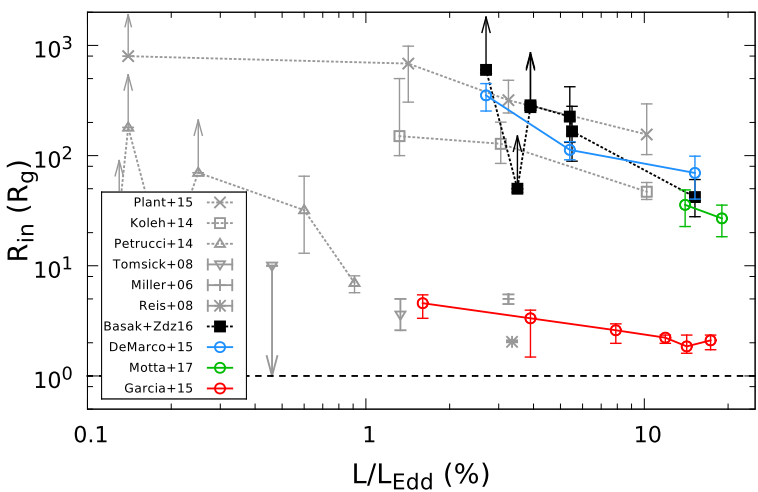
On the other hand, there has been an ongoing dispute regarding the nature of the hard state. The source has to be capable of emitting hard X-rays, which dominate the energetics in this state. A likely radiative process is Compton scattering by a predominantly thermal plasma, given an excellent description of the hard X-ray spectra by it, e.g., Gierliński et al. (1997). A lot of work has been devoted to the model in which this plasma forms a hot accretion flow surrounded by a cold outer disc (e.g. Shapiro, Lightman & Eardley 1976; Narayan & Yi 1995; Done, Gierliński & Kubota 2007; Yuan & Narayan 2014). Such a geometry (resembling a sombrero hat) is present in quiescence of BH transients and in initial stages of their outbursts (Lasota, Narayan & Yi 1996; Dubus, Hameury & Lasota 2001; Bernardini et al. 2016). In this model, it is natural to associate the transition to the soft state during a transient outburst with the viscous outer disc reaching the ISCO. This model does explain a lot of phenomena observed in BH binaries, e.g., correlations of the X-ray power-law index with the relative strength of Compton reflection, the broadening of the Fe Kα line, the characteristic frequencies in the power spectra, and the luminosity (e.g., Done, Gierliński & Kubota 2007; Gilfanov 2010, Gierliński; Zdziarski & Done 2011).
1.2 LIMITS OF THE LAMPPOST
Results have been controversial. In the case of GX 339–4, the same observations as those used by the authors claiming the disc extending to the ISCO have been fitted by others, who found the disc to be truncated (Done & Díaz-Trigo 2010; Kolehmainen, Done & Díaz-Trigo 2014; Plant et al. 2015; Basak & Zdziarski 2016), see Fig. 1. The differences have been explained by both instrumental effects (in particular, the instrumental pileup) and differences in modelling. For this source, a detailed comparison of different model results is given in Basak & Zdziarski (2016). In the case of the persistent BH binary Cyg X-1, Parker et al. (2015) claimed the disc extending to the ISCO, while Basak et al. (2017) have shown the same data can be fitted with a truncated disc.
In the case of the lamppost geometry, Niedźwiecki, Zdziarski & Szanecki (2016) have pointed out two major problems with its physical self-consistency in the case of the lamppost location close to the BH horizon. Due to light bending, only very few of the emitted photons are able to go to the observer; most either cross the horizon or hit the disc. This means the actual source luminosity (as measured at infinity) is much larger than that observed. Furthermore, the source luminosity as locally measured is even higher, due to the additional effects of time dilation and energy shift, which then implies that the source is completely out of equilibrium between production of e± pairs and their annihilation.
Furthermore, the nature of the transition between the hard and soft states becomes difficult to understand if the disc extends to the ISCO in both of them. The disc component in the hard state has a low maximum temperature, kTbb~0.1–0.2 keV, and its amplitude is weak, with the Comptonization luminosity being typically a factor of ~10 higher than that in the disc blackbody. This also means that the accretion rate within the disc in the hard state is much less than the total rate. The state transition would then correspond to the disappearance of the hot plasma and an increase of the disc accretion rate by a factor of a few tens (accounting also for the increase of the luminosity in the soft state) caused by an unspecified factor. No theory of such a process has been proposed yet. Also, the moment when the disc reaches the ISCO in transients, and the hot inner flow/outer disc configuration becomes replaced by a disc-corona geometry (at a luminosity much below that of the state transition) has to have no observational signature. This lack of an observational signature, in particular no change in the spectral index, is difficult to understand, given the radical change of the energy balance between the hot and cold components predicted in the two cases (e.g., Poutanen & Svensson 1996; Poutanen, Krolik & Ryde 1997; Zdziarski, Lubiński & Smith 1999; Poutanen, Veledina & Zdziarski 2018).
Finally, studies of the response of the blackbody component to changes in the hot component flux in the hard state of BH binaries show rather long time lags, corresponding to the distance between the disc and the bulk of the coronal emission of at least several tens of the gravitational radii (De Marco et al. 2015, 2017; De Marco & Ponti 2016). This is again consistent with the truncated disc geometry.
1.3 LIMITS OF THE SOMBRERO
On the other hand, the large number of detections of broad Fe lines during hard states constitutes a problem for the truncated disc model, as an inner disc is needed to account for the reflected photons. Below a few per cent of the Eddington luminosity, there is a general agreement about disc truncation. On the other hand, García et al. (2015) analysed a set of very high-sensitivity RXTE/PCA observations of GX 339–4, unaffected by the pile-up issues of CCD-based telescopes, and found an almost non-truncated disc above that luminosity. Miller et al. (2015) found a small inner radius in GRS 1739–278 using NuSTAR and argued for the presence of a compact corona. The robustness of these results shows that the evidence from reflection features is strong. While the precise measurement of BH spin need a detailed determination of the inner disc radius, these observations point towards a rather small inner radius.
Moreover, observations of the hard state of selected systems with low interstellar absorption have allowed the direct detection of a disc component, whose inner radius can be estimated from the best fit parameters. The disc inner temperature was low, but evidence for a small inner radius was found in a number of sources, for instance during a very long stare of GX 339–4 with XMM-Newton and RXTE (Miller et al. 2006). However, it is unclear how the inner disc, irradiated by the strong central source can keep its temperature low.
Furthermore, Kara et al. (2019) found the reverberation time lags between the continuum-emitting corona and the irradiated accretion disc in MAXI J1820+070 were 6 to 20 times shorter than previously seen. The timescale of the reverberation lags shortens by an order of magnitude over a period of weeks, whereas the shape of the broadened iron K emission line remains remarkably constant. This argues for a reduction in the spatial extent of the corona, rather than a change in the inner edge of the accretion disc.
A theoretical model that may explain the presence of broad Fe K lines in the hard state is that of a small residual inner disc due to collapse of a hot accretion flow (Liu et al. 2007; Taam et al. 2008), present in addition to the outer cold disc. On the other hand, theoretical advances in the reflection modelling may lead to an increase of the measured disc inner radii. Recently, Tomsick et al. (2018) have shown that the measured inner radius in a Suzaku/NuSTAR observation of Cyg X-1 increases from very close to the ISCO to ~7 ISCO radii when the density of the reflecting disc is increased from 1015 cm−3 (characteristic for AGNs) to 1020 cm−3 (expected in X-ray binaries). These observations and theoretical models must then be reconciled with those from timing analysis and with a global spectral fitting of the continuum.
1.4 FAST TIME VARIABILITY: REVERBERATION MAPPING AND TOMOGRAPHY
An independent set of constraints on the source geometry comes from fast time variability. The reflected emission should respond to fast fluctuations in the X-ray source brightness, so that it is a lagged and smoothed version of the continuum light curve. The lag is related to the timescale for light to travel between the X-ray source and the reflecting disc, so could give a direct measure of the mean distance of the disc from the source. This lag timescale was first seen in a breakthrough observation of an AGN, 1H 0707–495 (Fabian et al 2009). The very short delay time of 30s measured for this source is clearly consistent with a very small size scale between the X-ray source and disc, as predicted in the models with the lamppost very close to the event horizon of a maximally spinning BH.
This reverberation lag has now been identified in multiple other accreting BHs, both supermassive (de Marco et al 2013; Kara et al 2015) and stellar-mass (de Marco et al 2015, 2017; Kara et al. 2019), and these very new techniques are starting to be better understood. There are two main issues which complicate deriving a robust size scale from the measured reverberation lag. Firstly, the reverberation signal sits on top of a continuum. There is no ‘clean’ bandpass which contains either only reflected or only continuum emission, and this dilutes the observed lags. Secondly, the continuum itself has intrinsic lags, probably indicating that the X-ray source spectrum varies with radius. Fluctuations propagating down through the accretion flow go from larger radii (softer spectrum) down to the inner region (harder spectrum), imprinting a complex pattern of intrinsic continuum lags which underlie the reverberation signal (e.g. Uttley et al 2014).
Additionally, the QPOs provide a further opportunity to constrain the geometry of the inner disc. Ingram et al (2016) discovered that the centroid energy of the Fe line in the XRB H 1743–322 varies systematically over the course of a QPO cycle. This constitutes the most robust evidence to date that the QPO originates from Lense-Thirring precession of the inner flow, since the model predicts that different azimuths of the disc should be illuminated over the course of a precession cycle, giving rise to a blue/red shifted iron line when the approaching/receding disc material is illuminated. This result can be exploited to perform tomographic mapping: inferring the accretion geometry from the QPO phase-dependent shape of the Fe line profile. The first application of this technique yields an inner disc radius of ~30 Rg for H 1743–322 in the hard state (Ingram et al 2017).
Our team consists of specialists in both continuum and reverberation lags and in QPO tomography. Our goal here is to reach consensus on how to derive robust constraints from the data.
2. Specific goal of the proposed team
The team members have been selected to cover different aspects of the studied problem, and, crucially, they belong to both of the two opposite camps. As far as we know, our meetings will be the first ever opportunity allowing for extended discussions and confrontation of the opposite views on the geometry of BH accretion; unlike the case of conventional workshops and conferences, where talks usually present the views of the speaker and subsequent discussions are necessarily short. All of the team members are very active researchers as well as their international scientific standing clearly places them at the top in the field of X-ray astrophysics. Furthermore, they are specialists in the theory of accretion, spectral analysis, timing, and analysis of X-ray data. Also, our team has an excellent gender balance (5-7), an important issue stressed in the ISSI Annual Call.
Our work plan and the specific goals are as follows. (1) Removing degeneracies from models. For example, the same spectral data can be fitted as a broad line on top of a single power-law or as a narrow line on top of a continuum with a soft excess. The same variable time lags can be interpreted as due to a moving disc inner radius or a corona moving up and down. Results of Fourier-resolved spectroscopy depend on whether only the amplitude of the components is allowed to vary or spectral pivoting is allowed. We will discuss ways to break those degeneracies. (2) Finding ways to combine spectral and timing data. Such an approach has been pioneered in recent papers by Mahmoud & Done (2018a,b) and Mahmoud, Done & De Marco (2019). We will discuss those as well as alternative spectral/timing models. (3) Explaining why the same data can sometimes be fitted with very different results in different papers. We will use the specific data sets for which this was the case, and will go step by step through the process of data analysis and find the causes of the discrepant results. (4) Determining the status of the measurements of spins of BHs with hard-state spectral data. (5) Testing GR and constraining its alternative theories is of major importance, and can be done with X-ray data. We will discuss for which spectral states of accreting BHs such studies are possible, what can be done with existing data, and what real constraints follow from papers on this issue already published. (6) Determining what kind of observations with existing and planned instruments could resolve the present controversy, and planning strategies for future observing campaigns. During our work on the above issues, we will use existing data from RXTE, XMM-Newton, Suzaku, Swift and NuSTAR.
3. List of expected output
Almost all of our team members have published papers closely related to the subject of this proposal in recent years, and it is certain that this intense activity will continue in the future. Those new papers will acknowledge the ISSI programme. In addition, we plan to prepare a review paper (probably in Space Science Reviews) summarizing the status of our understanding of the accretion geometry in the BH vicinity. If we do not reach consensus, this paper will present evidence for and against the disc extending to the ISCO in the hard spectral state, and discuss tests, models and observations that would allow us to conclusively resolve the controversy. This will include, e.g., applying new theoretical spectral and timing models to existing data and applying for new crucial observations. Still, we hope that after our second meeting we will have a much clearer picture than now of the actual geometries of accretions flows onto BHs close to their horizons for different accretion rates and BH masses.
4. Features making ISSI the preferred site
The proposing team is distributed over six different countries. Many collaborations between team members are already taking place, but not on a full team scale. A global collaboration of this fashion can only be made possible by physical meetings of the members. Participation in a workshop centred on discussions is the ideal setting for it. In particular, a major advantage of ISSI is to provide a working/meeting external environment that allows team members to concentrate almost exclusively on the meeting topics and interactions with other members, removing them from other duties. We ask ISSI to fund two team meetings, each of them one week long, separated by one year. The first meeting should take place in the second half of 2019.
References
Basak, R., Zdziarski, A.A., 2016, MNRAS, 458, 2199
Basak, R., Zdziarski, A.A., Parker, M, Islam, N., 2017, MNRAS, 472, 4220
Bernardini F., et al., 2016, ApJ, 818, L5
De Marco, B., et al., 2013, MNRAS, 431, 2441; 2015, ApJ, 814, 50; 2017, MNRAS, 471, 1475
De Marco, B., Ponti, G., 2016, ApJ, 826, 70
Done, C., Díaz-Trigo, M., 2010, MNRAS, 407, 2287
Done, C., Gierliński, M., Kubota, A., 2007, ARA&A 15, 1
Dubus, G., Hameury, J.-M., Lasota, J.-P. 2001, A&A, 373, 251
Dziełak, M., Zdziarski, A. A., Szanecki, M., De Marco, B., Niedźwiecki, A., Markowitz, A., 2019, MNRAS, in press
Fabian, A. C., et al., 2009, Nature, 459, 540; 2014, MNRAS, 439, 2307
Fürst, F., et al., 2015, ApJ, 808, 122
García, J. A., et al., 2015, ApJ, 813, 84; 2018, ApJ, 864, 25
Gierliński, M., et al., 1997, MNRAS, 288, 958; 1999, MNRAS, 309, 496
Gierliński, M., Zdziarski, A.A., Done, C., 2011, Italian Physical Soc. Conf. Proc. 103, 299
Gilfanov, M., 2010, in The Jet Paradigm, Springer, p17
Ingram, A., et al., 2016, MNRAS, 461, 1967; 2017, MNRAS, 464, 2979
Kara, E., et al., 2015, MNRAS, 446, 737; 2019, Nature, 565, 198
Keck, M. L., et al., 2015, ApJ, 806, 149
Kolehmainen, M., Done, C., Díaz-Trigo, M., 2014, MNRAS, 437, 316
Lasota, J.-P., Narayan, R., Yi, I., 1996, A&A, 314, 813
Liu, B. F., et al., 2007, ApJ, 671, 695
Mahmoud R. D., Done C., 2018a, MNRAS, 473, 2084; 2018b, MNRAS, 480, 4040
Mahmoud R. D., Done C., De Marco B., 2019, MNRAS, submitted
Martocchia, A., Matt, G., 1996, MNRAS, 282, L53
Miller, J. M., et al., 2006, ApJ, 653, 525
Motta, S., et al., 2014a, MNRAS, 437, 2554; 2014b, MNRAS, 439, L65
Narayan, R., Yi, I., 1995, ApJ, 452, 710
Niedźwiecki, A., Zdziarski, A. A., Szanecki, M., 2016, ApJ, 821, L1
Niedźwiecki, A., Szanecki, M., Zdziarski, A. A., 2019, MNRAS, 485, 2942
Novikov, I. D., Thorne, K.S., 1973, in “Black Holes,” Gordon & Breach, Paris, 343
Parker, M. L., et al., 2014, MNRAS, 443, 1723; 2015, ApJ, 808, 9
Plant, D. S., et al., 2015, A&A, 573, 120
Poutanen, J., Svensson, R., 1996, ApJ, 470, 249
Poutanen, J., Vurm, I., 2009, ApJ, 690, L97
Poutanen, J., Krolik, J. H., Ryde, F., 1997, MNRAS, 292, L21
Poutanen, J.; Veledina, A.; Zdziarski, A. A., 2018, A&A,
Reis, R. C., Fabian, A. C., Miller, J.M., 2010, MNRAS, 402, 836
Rykoff, E. S., et al., 2007, ApJ, 666, 1129
Shakura, N. I., Sunyaev, R. A., 1973, A&A, 24, 337
Shapiro, S. L., Lightman, A. P., Eardley, D. M., 1976, ApJ, 204, 187
Taam R. E., et al., 2008, ApJ, 688, 527
Tomsick, J. A., et al., 2008, ApJ, 680, 593; 2018, ApJ, 855, 3
Uttley, P., et al., 2014, A&ARv, 22, 72
Xu, Y., et al., 2018, ApJ, 852, 34
Yuan, F., Narayan, R., 2014, ARA&A, 52, 529
Zdziarski, A. A., Gierliński, M., 2004, Progress of Theoretical Physics Suppl., 155, 99
Zdziarski, A. A., Lubiński, P., Smith, D. A., 1999, MNRAS, 303, L11